Demonstrate the temperature dependence of the Hall sensor sensitive layer materi
Demonstrate the temperature dependence of the Hall sensor sensitive layer material, Hall Coe
Introduce
Future fusion power reactors will operate in a continuous mode, and the magnetic field of these reactors will be stable. The applicability of magnetic coil based diagnostics for measuring steady-state magnetic fields under fusion reactor conditions is limited by signal integrator drift. Therefore, dedicated diagnosis of steady-state magnetic fields will be an important component of the diagnostic device for fusion power reactors, including demonstration of fusion power reactor prototypes.
The ITER reactor will be equipped with a steady-state magnetic diagnostic device based on a Hall sensor with a bismuth sensitive layer.
The bismuth sensor was approved by the ITER final design review expert committee and started production by the Institute of Plasma Physics of the Chinese Academy of Sciences in IPP Prague.
Currently, Hall sensors are proposed to perform absolute measurements of steady-state magnetic fields as part of demonstrating magnetic diagnostics. Hall sensors will be helpful in measuring plasma current, plasma wall gaps, and local disturbances in the surface of magnetic flux near the wall.
This paper evaluates the operating temperature, size, and temperature dependence of Hall coe factors for bismuth (Bi), antimony (Sb), molybdenum (Mo), tantalum (Ta), gold (Au), copper (Cu), and platinum (Pt) sensitive layer materials.
Hall Coe City
Hall coefficent is one of the main parameters considered for any sensitive material used in Hall sensors. The Hall voltage is proportional to the component of the magnetic field B perpendicular to the sensitive layer:
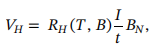
Where RH represents the Hall coefficient as a function of temperature t and magnetic field b, I is the sensor power supply current, BN is the normal component of the magnetic field, and t is the thickness of the sensitive layer. The size of the Hall Coe factor is always a function of temperature. Hall coefficient has an important impact on the sensitivity of the sensor to the magnetic field SH:
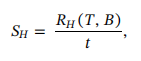
Higher Hall coefficent is a prerequisite for higher sensor sensitivity, and higher sensor sensitivity is a prerequisite for higher sensor output signals. In a fusion reactor environment, the size of the output signal is crucial for achieving measurement accuracy and noise suppression.
Experimental device
The test was conducted in the IPP Hall Sensor Laboratory. The stainless steel high vacuum chamber is equipped with a miniature ceramic resistance heater with a power of 40 W. The test magnetic field is generated by a pair of strong permanent magnets that provide a stable magnetic field of 270 mT at the sensor location. Figure 1 shows the sensor design before en.
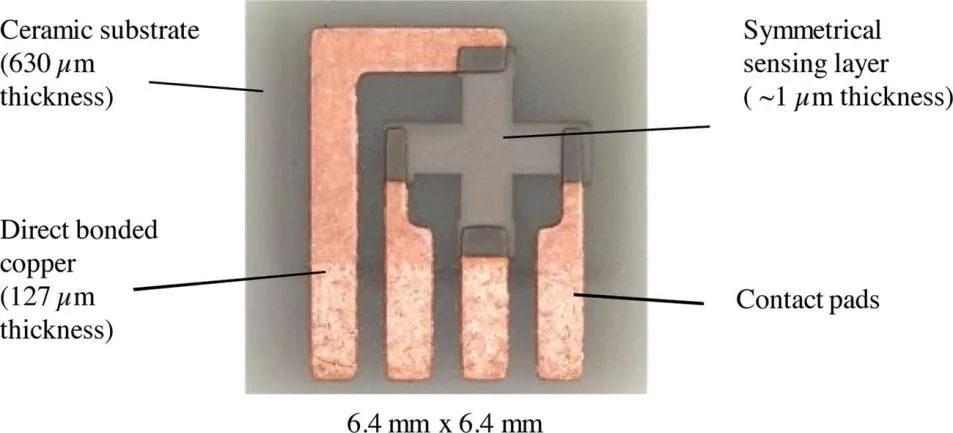
Figure 1. Design of measured Hall sensor
Result
The size of Hall Coe factors and their relationship to temperature are shown in Figure 2 on a logarithmic scale. Bismuth provides the highest Hall Coe content, followed by antimony, followed by molybdenum, tantalum, gold, copper, and platinum.
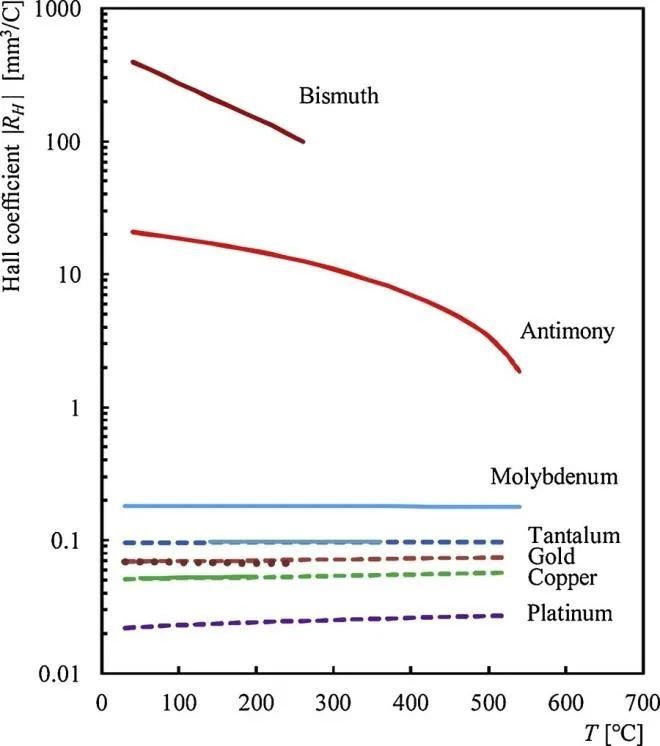
Figure 2. Comparison of all listed Hall Coes on a logarithmic scale
The attractiveness of tantalum as a candidate material lies in its extremely high melting temperature (3017 ° C) and the extremely low temperature dependence of sensor sensitivity. Unfortunately, the sensor output signal is very low, only half that of molybdenum. Preliminary results for the IPPT1 series of tantalum sensors were obtained at temperatures up to 360 ° C. The preparation technology of tantalum Hall sensors also needs further development.
Discuss
In order to evaluate the impact of temperature dependence on measurement accuracy, it is appropriate to normalize the Hall coefficients at room temperature (Figure 3). Assuming that the operating temperature variability during reactor pulses is 5 ℃, Table 1 shows the different results of temperature changes causing Hall coefficients to change, resulting in sensor output voltages below 0.1% in the case of tantalum and molybdenum.
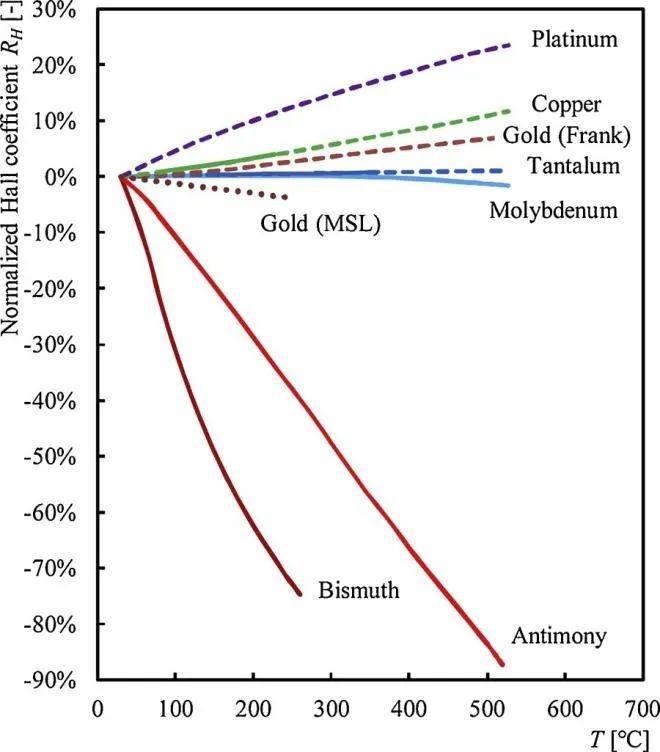
Figure 3. Relative changes in Hall Coefficients over the operating temperature range
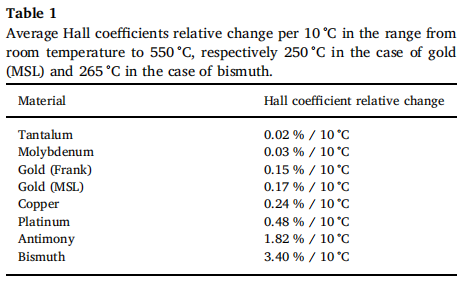
The sensitivity of currently available sensors is shown in Figure 4. The gold sensor developed by MSL has a relatively high sensitivity compared to other tested sensors due to its very thin sensing layer of 50 nanometers. The two presented sensitivities of the tantalum sensor correspond to different sensitivity layer thicknesses of 190 nm and 750 nm, respectively.
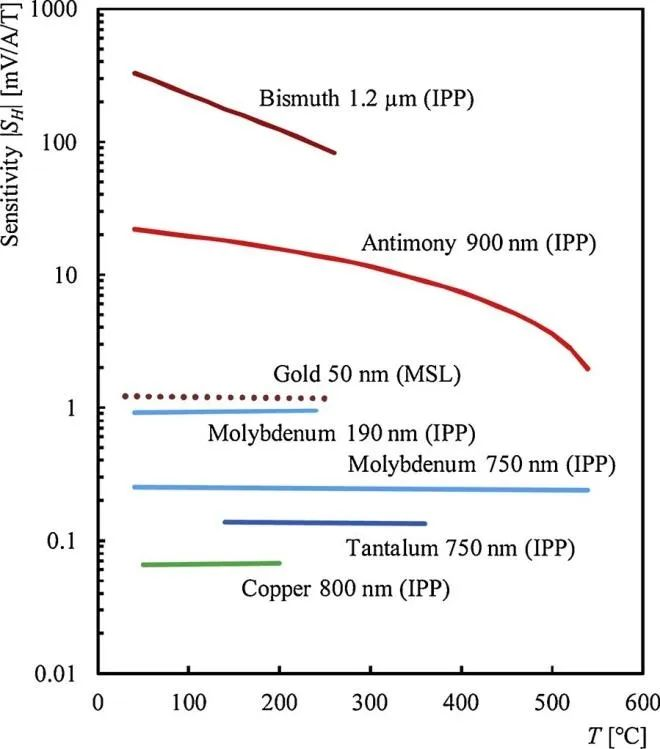
Figure 4. Sensitivity of a Hall sensor tested on a logarithmic scale in a demonstration project
Conclusion
Bismuth and antimony provide relatively high Hall coe factors, several orders of magnitude higher than other candidate materials, but these coe factors are highly temperature dependent, and their implementation requires dedicated sensors to measure the temperature of the sensor. The Hall Coefficients of molybdenum and tantalum are almost temperature independent. For the other materials considered here, the apparent temperature dependence of Hall Coe elements may require temperature measurements similar to those of bismuth and antimony.
The results show that bismuth and antimony have the best sensor sensitivity, while tantalum and molybdenum have the best output signal temperature stability.